Fish welfare and –performance in recirculation plants
By Bendik Fyhn Terjesen1, Jelena Kolarevic1, Grete Bæverfjord1, Harald Takle1,2 1Nofima, 6600 Sunndalsøra and 1432 Ås. 2 AVS Chile, Puerto Varas, Chile
The running costs in RAS, like in other types of plants, are, on the one hand, dependent on how effective the technology can deliver a given water quality, while on the other hand, it is also important that we actually know what water quality and other running conditions are important for good fish welfare, health and performance. In this article, we will focus on these last main factors, since relatively little information is available on which physiological, nutritional and health requirements the salmon has in an RAS environment.
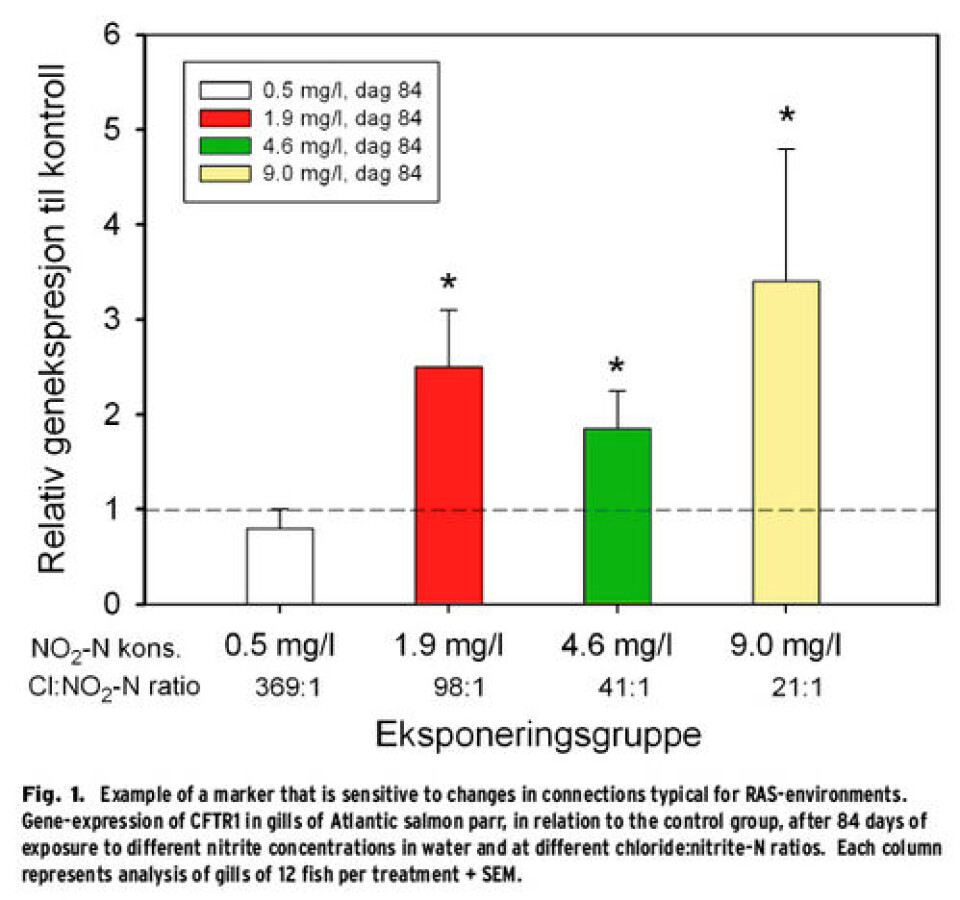
Comparison between RAS and flow-through It is possible that the use of recirculation of water throughout the smolt phase of Atlantic salmon yields a stable water quality and temperature, compared to traditional flow-through (FT), and through this it will have an effect on growth, physiology and welfare. Directly comparative studies of fish in recirculation and flow-through have, on the other hand, not been performed. Such studies are important in order to uncover both possible negative and positive effects of RAS on the fishes’ performance and welfare. In our experience it is important that under such controlled experiments one should have careful monitoring of the water temperature in the experiment, since pump- and pipe friction in the RAS will increase the temperature, even when the same water source is being used for both RAS and FT.
In an experiment performed at Nofima centre for recirculation in aquaculture (NCRA), at Sunndalsøra, salmon parr (7.1±0.1g ind-1, average ±SD) were put in four 3.2m3 tanks connected to a recirculation system (RAS), and four corresponding tanks that received flow-through water (FT), from the same source as the supply water of the RAS. The water temperature was kept equal in the two systems by using a district heating system to adjust the FT temperature (to 13.9±0.7ºC) to follow the RAS temperature (13.8±0.8ºC).The fish were light controlled until smoltification, and 110 days after the start of the experiment the smolt was set in sea water at Nofima Averøy (three experimental nets per treatment). After putting them in sea water, the feed uptake and growth of the fish were measured for 5.5 months. The growth rate in the freshwater phase, calculated for the whole period, did not show any significant effect of the water treatment (average 2.4±0.04% day-1), and neither were there any differences in the final weight after the sea phase (average 418±31g ind-1). The significance of controlling the temperature in order to be able to compare the results was shown in another experiment, which was performed at NCRA at the same time, but with different fish. Here, the FT-water was not warmed relative to the RAS-water (here 8.6±0.2ºC and 13.7±1.6ºC, respectively). In this case, the final weight at vaccination was much higher for the RAS fish (97 g ind-1) compared to the FT fish (46g ind-1). In combination, these experiments show that 1) RAS in itself increases the water temperature and thereby results in higher end weight and 2) it is important when doing such comparisons to monitor and or control the temperature. Even though the growth rate did not change as a result of treatment in the experiment with equal temperature, the experiment showed some other interesting differences. The mortality until launching in sea water was very low in both experimental treatments, but still, significantly higher for the RAS fish (1.0±0.4%), compared to the FT fish (0.4±0.1%). However, this result was only related to the first eight days after vaccination, while the mortality otherwise was not significantly different for the two treatments. After launching the fish in sea water there was no difference in survival between the two experimental groups. The first sea water test (34‰, 72hours) after completion of 12D:12L light steering showed that the RAS fish had significantly lower plasma chlorine concentrations than the FT fish after exposure to sea water, but this was equalized at the second and third sea water test before exposure to sea water. Preliminary results from molecular analyzes indicate that smolt produced in RAS has a different course in gene expression of ion transport such as Na +-K +-ATPase a1a / a1b, CFTR1 and NKCC1 compared with fish from FT. Analysis of the carbonate system in the blood showed that RAS fish had a significantly lower concentration of HCO3-than the FT-fish, which may indicate exposure to higher CO2 concentrations in the FT-water, or that FT-fish will respond differently from RAS fish to the same CO2 level. Analysis of external welfare markers showed that RAS-fish several times during the experiment had a significantly lower proportion of fin erosion and gill lid shortening, which may indicate improved welfare in the RAS, despite the fact that the consumption of fresh water in the RAS system was down to 2% of the FT plant.
How can we measure the fishes’ response? The fishes’ health and performance is controlled by a number of biological and non-biological factors and optimization of these can be very profitable for the fish farmers. In traditional, land based flow-through systems it has been common to concentrate on abiotic factors such as temperature and water quality. The complexity increases in RAS and optimisation of factors such as pH, ammonia/ammonium distribution, nitrite and CO2, in addition to control of water flow and –speed are important in order to meet the demands of increased economical profit and performance of the fish. In order to succeed with optimisation in the smolt production it is thus not enough only to use traditional and at times imprecise measuring criteria such as success of smoltification, growth and mortality. It has thus been important to develop new analyses that are tied up directly to the fishes’ response to e.g. ammonia, nitrite and CO2, in order to be able to give advice about tolerance levels and estimates of optimal conditions in different parts of the production cycle. Nofima has worked interdisciplinary to identify and characterize different molecular-physiological responses and markers in a toolbox that can be used in monitoring and optimization of the welfare and performance in RAS. Two examples of this toolbox are Rhesus glycoprotein 1 (Rhcg1) and cystic fibrosis transmembrane conductance regulator 1 (CFTR1), which we have found to respond very well to ammonia and nitrite, respectively, in the water (for Rhcg1, see Terjesen et al. (2010) and Kolarevic et al. (2012); for CFTR1, see Fig. 1).
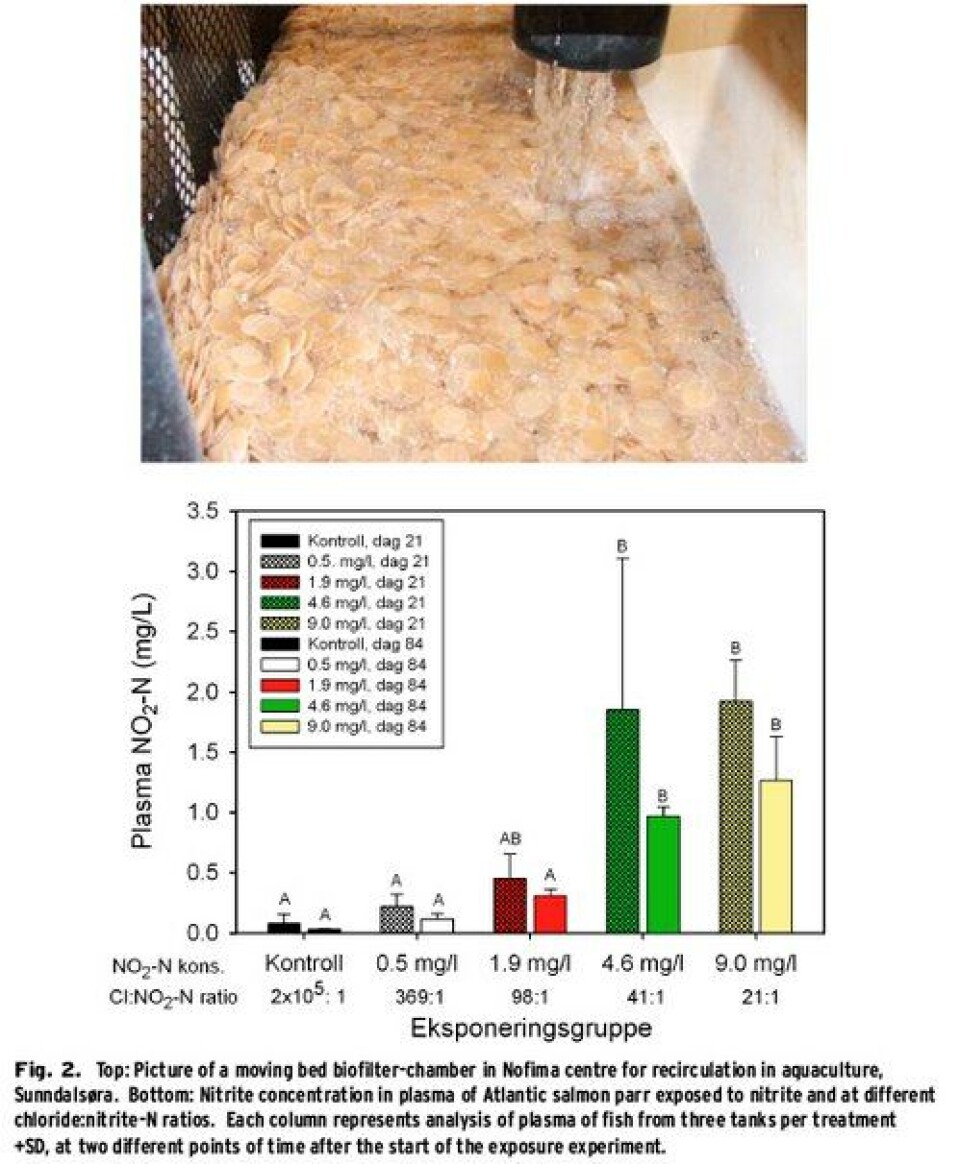
Nitrite, a problem with uneven operation of recirculation plants In well-funtioning biofilters (Fig. 2, top), nitrite (NO2) is converted quite quickly to nitrate( NO3). However, once the environmental- or running conditions in RAS become sub-optimal for effective nitrification, this can lead to rapid accumulation of nitrite. Reasons for this could be, e.g. big variations in feed dispensing from day to day; the variation should not be more than 15% from one day to the other (Emparanza, 2009). Negative effects of nitrite on fish are oxidation of haemoglobin to methemoglobin, and nitrite can disturb ion regulation and cardiovascular, endocrine and excretory processes in the fish. NO2-ions can substitute chloride (Cl-) in Cl-/HCO3-transporters in the gills and addition of NaCl to the water can thus reduce the fishes’ uptake of nitrite, since Cl- will then outperform NO2-. However, only limited amounts of information are available about the effect of longtime nitrite exposure of Atlantic salmon parr and which Cl:NO2-ratio (weight:weight) should be used in order to achieve protection of the fish. Parr (16.5±0.6 g ind-1, ±SD) were divided into several groups: either control treatment (no nitrite added) or one of four different nitrite concentrations and exposed for 12 weeks to either 0.00±0.00 (control), 0.53±0.21, 1.94±0.41, 4.60±0.56 or 8.99±1.06 mg l-1 NO2-N. The experiment was run in triplicate 150l tanks per treatment, supplied by groundwater in a flow through mode. NaCl was added in order to keep the Cl-level at 186±20mgl-1 in the treatments, which resulted in Cl:NO2-N ratios of 21:1, 41:1, 98:1 369:1 and up against infinity for the control group. No mortality related to treatment was observed during the experiment and the individual weight at the end of the experimental period was 80.9±8.4 g ind-1. However, a significantly reduced growth rate (SGR) was observed during the first three weeks for the group exposed to Cl:NO2-N ratio of 21:1. The concentration of nitrite in the plasma was significantly higher in the groups exposed to Cl:NO2-N ratios of 21:1 and 41:1 than in the control fish. The expression of CFTR1 in the gills showed a significant effect of nitrite exposure, as is shown in Fig. 1. Further studies of the interactions between nitrite and other water quality parameters are needed in order to be able to recommend Cl:NO2-N ratios for RAS. However, the experiment shows that under the tested conditions one can ensure growth and prevent nitrite accumulation in the fish and thus probably avoid damage to the blood by using a Cl:NO2-N ratio of >100:1. These findings show the necessity for defining specific guidelines for the water quality parameters for RAS, both, in relation to operations during smoltproduction, but also as a basis for the design and dimensioning of new systems (Terjesen et al., 2012).
Monitor the speed of water in recirculation systems In RAS, the dimensioning of water treatment can require relatively fast circulation of great quantities of water in tanks and in the whole system, especially when the efficiency of removal of e.g. TAN and CO2 are relatively low. This can lead to high speeds of water in the fish tanks. The question then is whether the speed of water can be too high and whether this is a factor that should be controlled. Previous research has shown that salmon in fresh water have the advantage of a certain swimming speed, since this gives both better growth and increased resistance against disease (Castro et al., 2011; Takle and Castro, 2013). However, the RAS environment is quite different from the FT one and we wanted to find out whether it was necessary to regulate the speed of water in RAS tanks, and identify the effects of high vs. low speed of water. Salmon parr were exposed for three different speeds of water – Low (LIC), Medium (MIC) and High (HIC) from the start of the photoperiod till smoltification and sea launching. In addition, the MIC and HIC groups were split up at the transition to continuous light and half of the fish were put in new tanks with water speeds of LIC, in order to test for the effects of low speeds of water during the last weeks before sea launching. Some of the fish in each group were marked individually, pooled and put in a common netpen at sea. In addition, some of the fish were sent to an infection test with Moritella viscosa, a bacterium associated with winter ulcers. The results showed that the highest speeds of water gave significantly higher weight, both during exposure and after 90 days at sea. In the Moritella-infection test, on the other hand, the group with the highest water speeds performed weakest (Fig.3), and the effect was the same even when the speed of water was reduced during the last weeks before sea launching. Our preliminary conclusion is that the water speed in RAS should be more than 1 body length s-1 in order to exploit the training concept for better growth, but not more than 1.5 bodylengths s-1 so that the resistance to disease does not get reduced. We are continuing our studies in order to find out physiological and molecular explanations for why the higher speed of water resulted in higher mortality because of winter ulcers. Recommendations for density of salmon parr in RAS Profitable operations in a RAS require high productivity and high fish density can be one means to achieve this. However, too high fish density can be counterproductive because of reduced fish welfare and growth and increased mortality. The effects of high fish density in RAS have complex causes, where, amongst others, the system’s sustainability for water quality, feed allocation and social interactions between fish play a role. Only limited information is available about the effects of fish density of salmon parr in RAS environments. In a recently completed experiment with big parr (start weight 80 g ind-1) we thus followed the fish with the aim of finding out where the limits for fish density in RAS are. The experiments were performed in triplicate tanks for each of the four treatments and they were started with two different densities (30 and 60 kg m-3, respectively). The groups were further divided into two temperature regimes, with a constant difference of at least 2ºC. The fish were monitored over a period of 10 weeks, until a minimum of twice the weight and density had been achieved. As far as fish welfare was concerned, it was shown that the combination of high temperature and high density was critical. We observed strong reactions of the fish at ~120-130 kg m-3 at 15ºC, with obvious stress reactions, increased mortality, low growth, cataract, strong fin erosion and increased size of heart and liver. On the other hand, we still found growth at ~120-130 kg m-3 in the group that had a lower water temperature, about 13ºC. The first signs of deviation were observed when the density was higher than 100 kg m-3, and the higher the water temperature, the lower the expected margin for getting negative effects will be. Preliminary analyses indicate that the water quality was not one of the limiting factors in our experiment. Based on the measured parameters in the experiment (amongst other O2, pH, NH3-N, NO2-N, NO3-N, alcality, CO2), the water quality was relatively good even at the end of the experiment in the group with the highest temperature and density (16 mg l-1 CO2). It is possible that the direct course of the increased mortality in the group with high temperature and density were acute stress and circulation failure. The preliminary conclusion is that, given good feed allocation and water quality, salmon parr in RAS can tolerate densities of up to 100 kg m-3. It is important to bear in mind that the density is increasing rapidly from day to day and that the system is operated in such a way that the density does not get too high. The results also showed that it is especially important to regulate the density when the water temperature exceeds 14°C and that it is important to monitor abnormal behaviour under such conditions.
Further research on physiological, health- and nutritional needs of salmon in RAS environments Our knowledge about what salmon can and cannot tolerate can be challenged in RAS. In the NFR-project ‘Fish welfare and performance in recirculation aquaculture systems’ (RASALMO) we studied some of the problems of importance for fish welfare and traits of fish performance and some of these are described above. Further studies should focus on combinations of different stressors in RAS (e.g. high nitrite and ammonia), CO2 tolerance in RAS and the significance for salmon of the composition of the microbial fauna in RAS. The smolt production is undergoing a change again, after the authorities have introduced a new rule on trial, where the fish can grow until 1kg before they are set out in traditional nets at sea. Use of recirculation systems and semi-closed systems at sea can be ways to achieve this. In order to be able to produce post-smolt of 1 kg, one will need new knowledge about what kind of environment this fish needs. Research is being done in this field in sea water RAS in NCRA at Sunndalsøra and at other partners in the NFR-project consortium ‘Optimalised post-smolt production’,OPP.
Reference list can be obtained when contacting the authors.
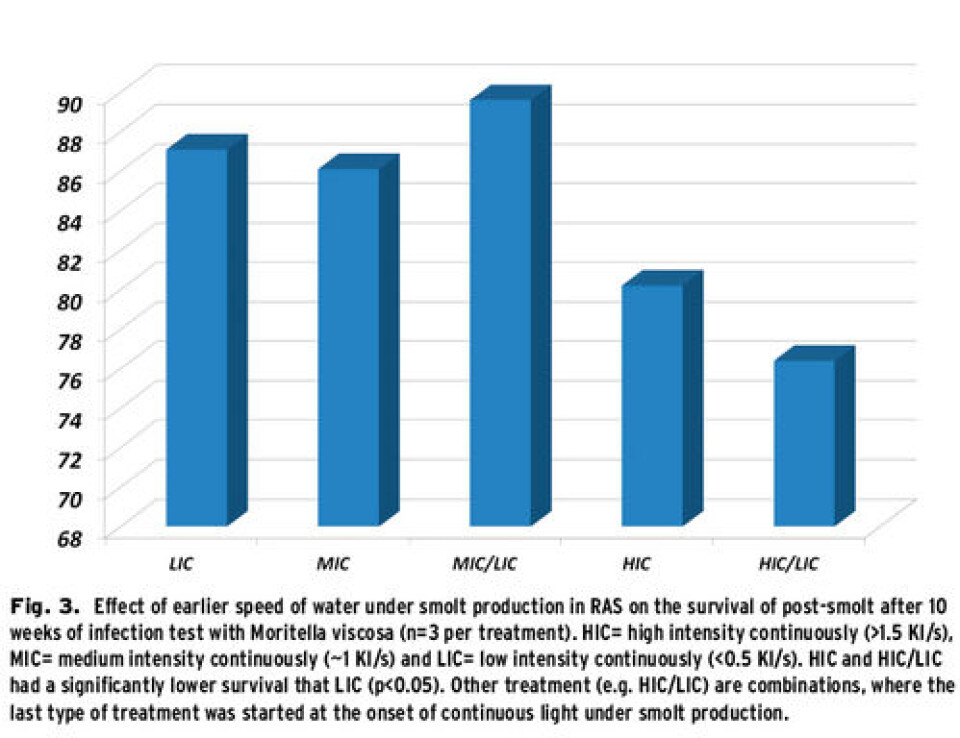